Welcome to the ultracold!
Ultracold quantum gases provide unique means to study quantum phenomena and exotic states of matter. Such gases combine a high degree of control over all relevant degrees of freedom, with the ability to precisely control their static and dynamic properties. They can thus serve as model systems to study many open questions from fundamental quantum mechanics to condensed matter physics. We routinely create these gases by cooling dysprosium atoms close to absolute zero temperature.
Physicists from Stuttgart prove the existence of a supersolid state of matter
Dipolar interactions
The behavior of ultracold atomic gases crucially depends on the interatomic interactions, which are typically described in terms of a simple contact interaction. This contact interaction is isotropic and short-ranged and thus akin to the interaction of colliding billiard balls. While this allows interesting insights, many systems in nature feature interparticle interactions that are more complex. This is the reason why in this project, we are interested in dipolar quantum gases, which additionally are subject to long-range magnetic interactions.
New states of matter
These magnetic interactions are not only long-ranged but can also be attractive or repulsive, depending on the orientation between the magnets. Based on this, already classical magnetic ferrofluids can show many interesting phenomena, such as self-organization and spontaneous symmetry breaking. Ultracold magnetic gases bring these phenomena into the quantum world. In the past few years, our experiment has allowed us to explore some of these exciting phenomena, such as dipolar Bose-Einstein condensates and new states of matter like self-bound quantum droplets and supersolids.
Research Highlights
In 2005, we produced the first Bose-Einstein condensate (BEC) of chromium atoms. This was the first example of a BEC with a non-negligible dipolar interaction. While in the initial experiments the dipole-dipole interaction was still only a small perturbation compared to the usual contact interactions, only a few years later a purely dipolar BEC was achieved by lowering the contribution of the contact interaction using a so-called Feshbach resonance. In this setting, the anisotropic nature of the dipole-dipole interaction led to a trap geometry dependent stability diagram, and a dipolar collapse with an anisotropic d-wave symmetry.
Since 2014, we are working with a new atomic species and are able to create quantum gases of dysprosium atoms. Dysprosium belongs to the group of rare-earth lanthanides and has the highest magnetic moment of all atoms in the periodic table. Compared to the usual alkali-metal atoms used in other BEC experiments, the dipole-dipole interaction between dysprosium atoms is enhanced by a factor of ~100. Therefore, dysprosium atoms are ideally suited to study the dipolar phenomena.
In order to create degenerate gases of dysprosium atoms, the atoms first have to be evaporated at about 1200°C in a high temperature effusion cell. The atoms in the resulting hot atomic beam are cooled with a Zeeman slower and subsequently further cooled and trapped in a magneto-optical trap (MOT). The cold temperature of a few micro Kelvin in our narrow-line MOT directly allows us to transfer the atoms into an optical dipole trap (ODT) in which quantum degeneracy can be achieved by evaporative cooling of the atoms. Using this experimental sequence, we can generate quantum gases with up to 50.000 atoms. These degenerate quantum gases then form the starting point for our experiments.
- Griesmaier et al., "Bose-Einstein condensation of chromium",
Phys. Rev. Lett. 94, 160401 (2005). - Lahaye et al., "Strong dipolar effects in a quantum ferrofluid",
Nature 448, 672 (2007). - Lahaye et al., "d-Wave Collapse and Explosion of a Dipolar Bose-Einstein Condensate",
Phys. Rev. Lett. 101, 080401 (2008).
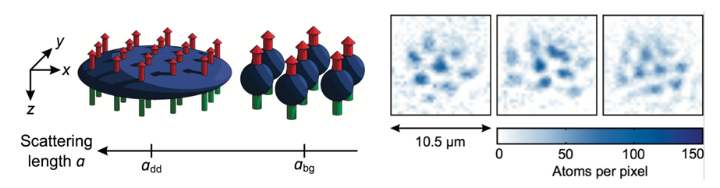
In 2015 we discovered the self-organization of our dipolar BEC into an array of smaller droplets. This behavior was very similar to the Rosensweig instability observed in classical ferrofluids. However, our observation came unexpected, as all theories available at the time predicted a collapse of the BEC. Nevertheless, these droplets remained stable and could be observed over several hundreds of milliseconds in our apparatus. Based on our observation, it was shown theoretically and experimentally shortly afterwards that the observed droplets are stabilized by quantum fluctuations. Such fluctuations are a result of the Heisenberg uncertainty principle. They are a very small effect that can typically be neglected. In dipolar quantum gases, they can nevertheless play a decisive role because of the complex interplay of contact and dipolar interactions.
- Kadau et al., "Observing the Rosensweig instability of a quantum ferrofluid",
Nature 530, 194 (2016). - Böttcher et al., "New states of matter with fine-tuned interactions: quantum droplets and dipolar supersolids",
Reports on Progress in Physics 84, 012403 (2021).

Similar to classical liquids, in which the liquid properties arise from the interplay between attractive inter-particle interactions and a strong short-range repulsion due to the Pauli principle, quantum droplets exhibit liquid-like properties due to the interplay between attractive mean-field interactions and repulsive quantum fluctuations. The most striking consequence is the self-bound nature of these quantum droplets. As a gaseous phase, a typical BEC needs a container to prevent it from expanding indefinitely. In contrast to this, quantum droplets constitute a self-bound state that remains bound even in the absence of an external confinement. They thus behave like a liquid, allthough they are more than a million times more dilute. In the experiment, we were able to prove their self-bound nature by observing levitated quantum droplets for up to 100 milliseconds even without the presence of a trap.
- Schmitt et al., "Self-bound droplets of a dilute magnetic quantum liquid",
Nature 539, 259 (2016). - Ferrier-Barbut et al., "Observation of quantum droplets in a dipolar Bose gas",
Phys. Rev. Lett. 116, 215301 (2016).
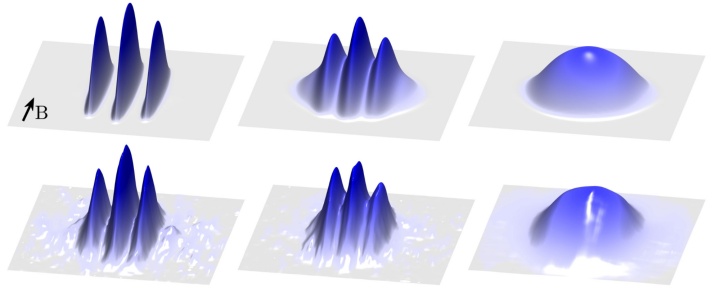
In 2019, based on our previous discovery of stable quantum droplets, we succeeded in proving experimentally that the long-sought supersolid state of matter exists. A supersolid is a counterintuitive phase of matter, which simultaneously features both the frictionless flow of a superfluid, as well as the crystalline structure of a solid.
The definitive proof that the regular patterns observed in the experiment form indeed a supersolid relies on our observation of two kinds of sound waves. Such sound waves propagate differently in different materials – in air, for example, sound waves travel much slower than in water. This "normal" sound wave is also present in the supersolid. However, because the supersolid is both solid and fluid, a characteristic second form of sound wave can be observed, in which the crystal and the superfluid move against each other. This results in characteristic sound waves (so called low-energy Goldstone modes) that travel at very low speeds, which we were able to observe for the first time in our experiment.
Furthermore, we were able to investigate the nature of the superfluid-supersolid phase transition by looking at the dynamical emergence of density fluctuations in the system. These density fluctuations are dominated by the roton excitations, which are periodic density modulations on top of the BEC. The softening of these roton excitations eventually trigger the crystallization process that forms the supersolid crystal. In the future, we plan to investigate the possibility of creating a supersolid where the droplets self-assemble in two dimensions. The increased complexity of this higher dimensionality offers the possibility of discovering many more new states of matter.
- Böttcher et al., "Transient Supersolid Properties in an Array of Dipolar Quantum Droplets",
Phys. Rev. X 9, 011051 (2019). - Guo et al., "The low-energy Goldstone mode in a trapped dipolar supersolid",
Nature 574, 386--389 (2019). - Hertkorn et al., "Density Fluctuations across the Superfluid-Supersolid Phase Transition in a Dipolar Quantum Gas",
Phys. Rev. X, in print (2021).
We are currently also setting up a next-generation experiment for the production of quantum degenerate dysprosium gases. With this new experimental setup, the production of significantly larger BECs will become possible, thus allowing for larger droplet crystals and the study of more complex states of matter. In addition, we plan to implement an optical lattice in the near UV, which will lead to very strong dipole-dipole interactions between atoms on adjacent lattice sites. In combination with a novel quantum gas microscopy scheme based on a long-lived intermediate state in dysprosium, we will be able to resolve single atoms in the lattice with a single site resolution. This single site resolved imaging combined with long coherence times will allow us to study Fermi- and Bose-Hubbard model physics enriched by the long-range and anisotropic dipolar interactions.
Publications
Project Publications
2024
- J. Hertkorn, P. Stürmer, K. Mukherjee, K. S. H. Ng, P. Uerlings, F. Hellstern, L. Lavoine, S. M. Reimann, T. Pfau, R. Klemt, Decoupled sound and amplitude modes in trapped dipolar supersolids, Physical Review Research 6 (2024), doi:10.1103/physrevresearch.6.l042056.
2022
- T. Langen, Dipolar supersolids: Solid and superfluid at the same time, Physics Today 75, 36–41 (2022).
- L. Chomaz, I. Ferrier-Barbut, F. Ferlaino, B. Laburthe-Tolra, B. L. Lev, T. Pfau, Dipolar physics: a review of experiments with magnetic quantum gases, Reports on Progress in Physics 86, 026401 (2022).
- L. Chomaz, I. Ferrier-Barbut, F. Ferlaino, B. Laburthe-Tolra, B. L. Lev, T. Pfau, Dipolar physics: A review of experiments with magnetic quantum gases (2022), (available at http://arxiv.org/abs/2201.02672).
- J. Fraxanet, D. Gonzalez-Cuadra, T. Pfau, M. Lewenstein, T. Langen, L. Barbiero, Topological quantum critical points in the extended Bose-Hubbard model, Phys. Rev. Lett. 128, 043402 (2022).
2021
- F. Böttcher, J.-N. Schmidt, J. Hertkorn, K. S. H. Ng, S. D. Graham, M. Guo, T. Langen, T. Pfau, New states of matter with fine-tuned interactions: quantum droplets and dipolar supersolids, Reports on Progress in Physics 84, 012403 (2021).
- J. Hertkorn, J.-N. Schmidt, M. Guo, F. Böttcher, K. S. H. Ng, S. D. Graham, P. Uerlings, H. P. Büchler, T. Langen, M. Zwierlein, T. Pfau, Supersolidity in Two-Dimensional Trapped Dipolar Droplet Arrays, Physical Review Letters 127, 155301 (2021).
- J. Hertkorn, J.-N. Schmidt, F. Böttcher, M. Guo, M. Schmidt, K. Ng, S. Graham, H. P. Büchler, T. Langen, M. Zwierlein, T. Pfau, Density Fluctuations across the Superfluid-Supersolid Phase Transition in a Dipolar Quantum Gas, Phys. Rev. X 11, 011037 (2021).
- J.-N. Schmidt, J. Hertkorn, M. Guo, F. Böttcher, M. Schmidt, K. S. H. Ng, S. D. Graham, T. Langen, M. Zwierlein, T. Pfau, Roton Excitations in an Oblate Dipolar Quantum Gas, Phys. Rev. Lett. 126, 193002 (2021).
- J. Hertkorn, J.-N. Schmidt, M. Guo, F. Böttcher, K. S. H. Ng, S. D. Graham, P. Uerlings, T. Langen, M. Zwierlein, T. Pfau, Pattern Formation in Quantum Ferrofluids: from Supersolids to Superglasses, Physical Review Research 3, 033125 (2021).
2020
- M. Guo, T. Pfau, A new state of matter of quantum droplets, Frontiers of Physics 16, 32202 (2020).
2019
- F. Böttcher, M. Wenzel, J.-N. Schmidt, M. Guo, T. Langen, I. Ferrier-Barbut, T. Pfau, R. Bomb\’ın, J. Sánchez-Baena, J. Boronat, F. Mazzanti, Dilute dipolar quantum droplets beyond the extended Gross-Pitaevskii equation, Phys. Rev. Research 1, 033088 (2019).
- J. Hertkorn, F. Böttcher, M. Guo, J. N. Schmidt, T. Langen, H. P. Büchler, T. Pfau, Fate of the Amplitude Mode in a Trapped Dipolar Supersolid, Phys. Rev. Lett. 123, 193002 (2019).
- F. Böttcher, J.-N. Schmidt, M. Wenzel, J. Hertkorn, M. Guo, T. Langen, T. Pfau, Transient Supersolid Properties in an Array of Dipolar Quantum Droplets, Phys. Rev. X 9, 011051 (2019).
- M. Guo, F. Böttcher, J. Hertkorn, J.-N. Schmidt, M. Wenzel, H. P. Büchler, T. Langen, T. Pfau, The low-energy Goldstone mode in a trapped dipolar supersolid, Nature 574, 386--389 (2019).
- J.-F. Mennemann, T. Langen, L. Exl, N. J. Mauser, Optimal control of the self-bound dipolar droplet formation process, Computer Physics Communications 244, 205--216 (2019).
2018
- I. Ferrier-Barbut, M. Wenzel, F. Böttcher, T. Langen, T. Pfau, Onset of a modulational instability in trapped dipolar Bose-Einstein condensates, Phys. Rev. A 97, 011604 (2018).
- I. Ferrier-Barbut, T. Pfau, Quantum liquids get thin, Science 359, 274 (2018).
- I. Ferrier-Barbut, M. Wenzel, F. Böttcher, T. Langen, M. Isoard, S. Stringari, T. Pfau, Scissors Mode of Dipolar Quantum Droplets of Dysprosium Atoms, Phys. Rev. Lett. 120, 160402 (2018).
- M. Wenzel, T. Pfau, I. Ferrier-Barbut, A fermionic impurity in a dipolar quantum droplet, Physica Scripta 93, 104004 (2018).
- M. Wenzel, F. Böttcher, J.-N. Schmidt, M. Eisenmann, T. Langen, T. Pfau, I. Ferrier-Barbut, Anisotropic Superfluid Behavior of a Dipolar Bose-Einstein Condensate, Phys. Rev. Lett. 121, 030401 (2018).
- T. Langen, M. J. Mark, Ultrakalt magnetisiert, Physik Journal 17, 35 (2018).
2017
- M. Wenzel, F. Böttcher, T. Langen, I. Ferrier-Barbut, T. Pfau, Striped states in a many-body system of tilted dipoles, Phys. Rev. A 96, 053630 (2017).
2016
- H. Kadau, M. Schmitt, M. Wenzel, C. Wink, T. Maier, I. Ferrier-Barbut, T. Pfau, Observing the Rosensweig instability of a quantum ferrofluid, Nature 530, 194 (2016).
- I. Ferrier-Barbut, M. Schmitt, M. Wenzel, H. Kadau, T. Pfau, Liquid quantum droplets of ultracold magnetic atoms, J. Phys. B: At. Mol. Opt. Phys 49, 214004 (2016).
- I. Ferrier-Barbut, H. Kadau, M. Schmitt, M. Wenzel, T. Pfau, Observation of quantum droplets in a strongly dipolar Bose gas, Phys. Rev. Lett. 116, 215301 (2016).
- I. Ferrier-Barbut, Smashing magnets, New Journal of Physics 18, 111004 (2016).
- M. Schmitt, M. Wenzel, B. Böttcher, I. Ferrier-Barbut, T. Pfau, Self-bound droplets of a dilute magnetic quantum liquid, Nature 539, 259 (2016).
2015
- T. Maier, H. Kadau, M. Schmitt, M. Wenzel, I. Ferrier-Barbut, T. Pfau, A. Frisch, S. Baier, K. Aikawa, L. Chomaz, M. J. Mark, F. Ferlaino, C. Makrides, E. Tiesinga, A. Petrov, S. Kotochigova, Emergence of chaotic scattering in ultracold Er and Dy, Phys. Rev. X 5, 041029 (2015).
- T. Maier, I. Ferrier-Barbut, H. Kadau, M. Schmitt, M. Wenzel, C. Wink, T. Pfau, K. Jachymski, P. S. Julienne, Phys. Rev. A, in press, doi:10.1103/PhysRevA.92.060702.
2014
- T. Maier, H. Kadau, M. Schmitt, A. Griesmaier, T. Pfau, Narrow-line magneto-optical trap for dysprosium atoms, Optics Letters 39, 3138 (2014).
2013
- K. Pawlowski, P. Bienias, T. Pfau, K. Rzazewski, Correlations of a quasi-two-dimensional dipolar ultracold gas at finite temperatures, Phys. Rev. A 87, 043620 (2013).
- M. Schmitt, E. A. L. Henn, J. Billy, H. Kadau, T. Maier, A. Griesmaier, T. Pfau, Spectroscopy of a narrow-line optical pumping transition in dysprosium, Opt. Lett. 38, 637 (2013).
- A. Maluckov, G. Gligoric, L. Hadzievski, B. A. Malomed, T. Pfau, High- and low-frequency phonon modes in dipolar quantum gases trapped in deep lattices, Phys. Rev. A 87, 023623 (2013).
- P. Bienias, K. Pawlowski, T. Pfau, K. Rzazewski, Ground state of a two component dipolar Fermi gas in a harmonic potential, Phys. Rev. A 88, 043604 (2013).
- D. Peter, A. Griesmaier, T. Pfau, H. P. Büchler, Driving dipolar fermions into the quantum Hall regime by spin-flip induced insertion of angular momentum, Phys. Rev. Lett. 110, 145303 (2013).
2012
- D. Peter, K. Pawłowski, T. Pfau, K. Rzążewski, Mean-field description of dipolar bosons in triple-well potentials, J. Phys. B: At. Mol. Opt. Phys. 45, 225302 (2012).
- A. Maluckov, G. Gligoric, L. Hadzievski, B. A. Malomed, T. Pfau, Stable periodic density waves in dipolar Bose-Einstein condensates trapped in optical lattices, Phys. Rev. Lett. 108, 140402 (2012).
- J. Billy, E. A. L. Henn, S. Müller, T. Maier, H. Kadau, A. Griesmaier, M. Jona-Lasinio, L. Santos, T. Pfau, Deconfinement-induced collapse of a coherent array of dipolar Bose-Einstein condensates, Phys. Rev. A 86, 051603 (2012).
2011
- S. Müller, J. Billy, E. A. L. Henn, H. Kadau, A. Griesmaier, M. Jona-Lasinio, L. Santos, Stability of a dipolar Bose-Einstein condensate in a one-dimensional lattice, Phys. Rev. A 84, 053601 (2011).
2009
- J. Metz, T. Lahaye, B. Fröhlich, A. Griesmaier, T. Pfau, H. Saito, Y. Kawaguchi, M. Ueda, Coherent collapse of a dipolar Bose-Einstein condensate for different trap geometries, New J. Phys. 11, 055032 (2009).
2008
- A. Muramatsu, T. P. (eds.), Focus on Quantum Correlations in Tailored Matter, New J. Phys. 10, 045001 (2008).
- T. Lahaye, J. Metz, B. Fröhlich, T. Koch, M. Meister, A. Griesmaier, T. Pfau, H. Saito, Y. Kawaguchi, M. Ueda, d-Wave Collapse and Explosion of a Dipolar Bose-Einstein Condensate, Phys. Rev. Lett 101, 080401 (2008).
- T. Koch, T. Lahaye, J. Metz, B. Fröhlich, A. Griesmaier, T. Pfau, Stabilizing a purely dipolar quantum gas against collapse, Nature Physics 4, 218 (2008).
2007
- Th. Lahaye, T. Koch, B. Fröhlich, M. Fattori, J. Metz, A. Griesmaier, S. Giovanazzi, T. Pfau, Strong dipolar effects in a quantum ferrofluid, Nature 448, 672 (2007).
- L. Santos, M. Fattori, J. Stuhler, T. Pfau, Spinor condensates with a laser-induced quadratic Zeeman effect, Phys. Rev. A 75, 053606 (2007).
- J. Stuhler, A. Griesmaier, J. Werner, T. Koch, M. Fattori, T. Pfau, Ultracold chromium atoms: From Feshbach resonances to a dipolar Bose-Einstein condensate, J. Mod Opt. 54, 647 (2007).
- B. Fröhlich, T. Lahaye, B. Kaltenhäuser, H. Kübler, S. Müller, T. Koch, M. Fattori, T. Pfau, A two-frequency acousto-optic modulator driver to improve the beam pointing stability during intensity ramps, Rev. Sci. Instrum. 78, 043101 (2007).
- K. Glaum, A. Pelster, H. Kleinert, T. Pfau, Critical Temperature of Weakly Interacting Dipolar Condensates, Phys. Rev. Lett. 98, 080407 (2007).
- S. Giovanazzi, L. Santos, T. Pfau, Collective oscillations of dipolar Bose-Einstein condensates and accurate comparison between contact and dipolar interaction, Phys. Rev. A 75, 015604 (2007).
2006
- M. Fattori, T. Koch, S. Goetz, A. Griesmaier, S. Hensler, J. Stuhler, T. Pfau, Demagnetization cooling of a gas, Nature Physics 2, 765 (2006).
- L. Santos, T. Pfau, Spin-3 Chromium Bose-Einstein Condensates, Phys. Rev. Lett. 96, 190404 (2006).
- A. Griesmaier, J. Stuhler, T. Pfau, Production of a chromium Bose-Einstein condensate, Appl. Phys. B 82, 211 (2006).
2005
- J. Stuhler, A. Griesmaier, T. Koch, M. Fattori, S. Giovanazzi, P. Pedri, L. Santos, T. Pfau, Observation of Dipole-Dipole Interaction in a Degenerate Quantum Gas, Phys. Rev. Lett. 95, 150406 (2005).
- A. Griesmaier, J. Werner, S. Hensler, J. Stuhler, T. Pfau, Bose-Einstein condensation of chromium, Phys. Rev. Lett. 94, 160401 (2005).
- S. Hensler, A. Greiner, J. Stuhler, T. Pfau, Depolarisation cooling of an atomic cloud, Europhys. Lett. 71, 918 (2005).
2003
- S. Hensler, J. Werner, A. Griesmaier, P. O. Schmidt, A. Görlitz, T. Pfau, S. Giovanazzi, K. Rzazewski, Dipolar Relaxation in an ultra-cold Gas of magnetically trapped chromium atoms, Appl. Phys. B 77, 765 (2003).
We are looking for motivated new team members!
The 5th Institute of Physics is looking for students who would like to experience everyday work in a modern quantum optics laboratory.
A variety of techniques and skills will be used:
- CAD Modelling / 3D printing,
- electronics,
- usage of optic devices/laser,
- measuring techniques.
Previous experience with these techniques is an advantage but not a requirement.
Salary: regular Hiwi salary per hour, hours per week and schedule upon individual arrangement.
Interested candidates should contact Tilman Pfau.
Are you interested in studying novel and unexpected quantum phenomena in dipolar quantum gases? Our group is currently looking for students to join our team studying novel phases of matter, such as quantum droplets and dipolar supersolids.
We are currently setting up a new experimental setup that will allow us to create quantum degenerate systems with a significantly larger atom number. This will facilitate the realization of new exotic phases of matter, which are predicted to emerge at higher atom numbers than currently achievable in our setup. In addition, we are planning to study the effect of a large nearest neighbor interaction in lattice systems by implementing a UV optical lattice. By implementing a novel quantum gas microscopy scheme based on a long-lived excited state in dysprosium, we expect to attain single-site resolution in a system with a small lattice spacing of only ~180nm. With this we plan to study Fermi- and Bose-Hubbard model physics enriched by long-range and anisotropic dipolar interactions.
If you are interested in learning more, please contact Tilman Pfau.
Many-body physics with dipolar quantum gases
In this project you will study superfluidity and many-body phases in dipolar quantum gases. The project will involve experimental research using ultracold dysprosium atoms imaged under a high-resolution optical microscope. Part of the experimental work will be conducted at the University of Stuttgart, Germany, and part at Swinburne University of Technology, Melbourne, Australia. This unique scholarship opportunity is supported by an established Swinburne/Stuttgart joint PhD programme and will provide experiences working in two leading research laboratories.
Candidates will enrole at both participating universities and receive a stipend from Swinburne University for up to 3,5 years.
The thesis will be supervised by Prof. Tilman Pfau (Stuttgart), Prof. Christopher Vale (Swinburne), Dr. Sascha Hoinka (Swinburne).
Please contact Tilman Pfau (University of Stuttgart) and Christopher Vale (Swinburne University of Technology) if you are interested in this opportunity.
Please contact Tilman Pfau if you are interested in joining our team as a Postdoc!
Project Team
Project News
Contact
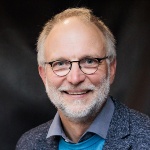
Tilman Pfau
Prof. Dr.Head of Institute